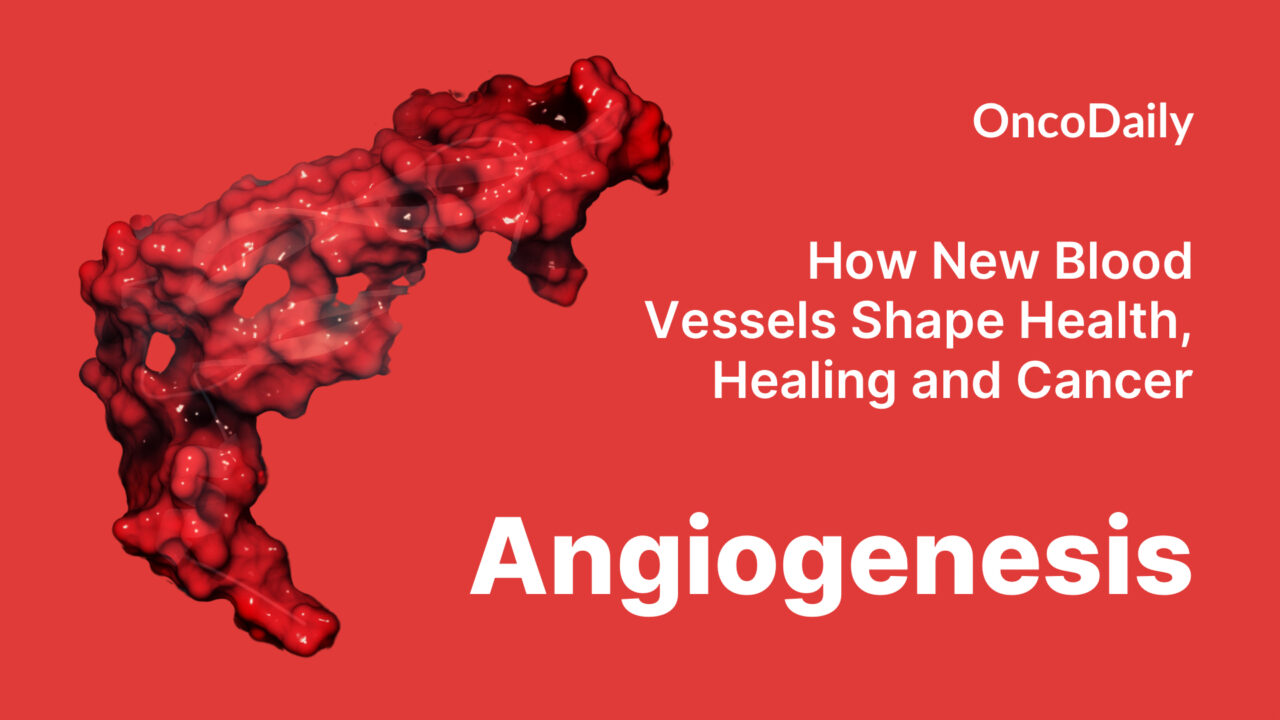
Angiogenesis: How New Blood Vessels Shape Health, Healing and Cancer
Angiogenesis is the biological process through which new blood vessels form from pre-existing ones. This intricate mechanism plays a fundamental role in growth, development, and healing by supplying tissues with oxygen and nutrients. It is tightly regulated in healthy individuals, turning on during wound healing or menstruation and turning off when no longer needed. However, when misregulated, angiogenesis can contribute to serious conditions such as cancer, diabetic complications, and chronic inflammation. In cancer, for instance, tumors exploit this process to build their own blood supply, allowing them to grow and spread.
In this article, we’ll explore the mechanisms that drive angiogenesis, the key molecules involved in its regulation, its role in both health and disease, and how modern therapies are designed to target or stimulate angiogenesis depending on the clinical context.
Why Angiogenesis Matters
Angiogenesis is essential for many normal biological functions throughout life. During embryonic development, it enables the formation of an entire vascular network, allowing the growing fetus to receive oxygen and nutrients. It also plays a key role in reproductive function, including the growth of the uterine lining during the menstrual cycle and the formation of the placenta during pregnancy. In adults, angiogenesis becomes especially important in tissue regeneration and wound healing. When tissues are injured, the body activates angiogenesis to restore blood supply, deliver immune cells, and support repair processes. Without this response, wounds would not heal properly, and damaged organs would struggle to recover.
However, angiogenesis must remain finely balanced. If it’s too active, it can lead to abnormal blood vessel growth seen in conditions like cancer, diabetic retinopathy, or psoriasis. If it’s insufficient, poor circulation and tissue ischemia can occur, contributing to chronic wounds and cardiovascular disease. This delicate balance is why understanding and controlling angiogenesis is so vital in both health and medicine.
Key Steps in the Angiogenesis Process
The process of angiogenesis unfolds in a series of coordinated steps, all regulated by a delicate balance between pro-angiogenic and anti-angiogenic signals. It begins when the body detects a need for new blood supply—for example, in response to tissue injury or oxygen deprivation. This triggers the release of chemical signals, most notably vascular endothelial growth factor (VEGF), which activates endothelial cells, the cells that line the inside of existing blood vessels.
Once activated, these endothelial cells begin to degrade the basement membrane, the thin layer of support that normally keeps them in place. Freed from this barrier, they migrate toward the area where new blood vessels are needed, guided by gradients of VEGF and other growth factors. As they reach the target site, the endothelial cells begin to proliferate and align, forming primitive capillary tubes that serve as the framework for new vessels. Over time, these structures become more stable through the recruitment of supporting cells called pericytes, which wrap around the vessel walls and help maintain their integrity and function.
Throughout this process, the body maintains a careful balance between signals that promote angiogenesis (such as VEGF, fibroblast growth factor, and angiopoietins) and those that inhibit it (such as thrombospondin, angiostatin, and endostatin). This regulation ensures that angiogenesis occurs only when necessary and does not continue unchecked—a critical safeguard against disease.
Molecular Regulators of Angiogenesis
Angiogenesis is tightly regulated by a dynamic interplay between pro-angiogenic and anti-angiogenic factors. These molecular signals ensure that blood vessels form when and where they’re needed—such as during growth, wound repair, or recovery from injury—while preventing unnecessary or harmful vessel formation.
Among the pro-angiogenic factors, the most critical is VEGF (vascular endothelial growth factor). VEGF directly stimulates endothelial cells to grow, migrate, and form new vessels. Other important players include FGF (fibroblast growth factor), which also promotes cell proliferation and tissue repair; angiopoietins, which help mature and stabilize blood vessels; and PDGF (platelet-derived growth factor), which recruits supporting cells like pericytes to the new vascular structures.
In contrast, the body produces anti-angiogenic factors to keep vessel growth in check. These include thrombospondin, angiostatin, and endostatin, which counteract pro-angiogenic signals and help ensure that new vessels form only when necessary. These inhibitors are especially important in preventing excessive or disorganized vessel growth that can lead to disease. One of the most powerful triggers for angiogenesis is hypoxia, or low oxygen levels. In response to hypoxia, cells activate HIF-1α (hypoxia-inducible factor-1 alpha), a transcription factor that increases the production of VEGF and other pro-angiogenic genes. This mechanism is vital during tissue repair and in tumors, where fast-growing cancer cells outpace their blood supply and use hypoxia signaling to stimulate new vessel formation and support their survival.
This complex network of signaling molecules highlights just how precisely angiogenesis is controlled—and how its disruption can lead to pathological conditions like cancer, retinopathy, or poor wound healing.
Angiogenesis in Cancer
In the context of cancer, angiogenesis becomes a crucial enabler of tumor survival and progression. In their earliest stages, tumors are limited in size—typically no more than 1 to 2 millimeters in diameter—because they lack access to their own blood supply. To grow larger and sustain rapid cell division, tumors must develop a vascular network to deliver oxygen and nutrients and to remove waste products. This is where tumor angiogenesis comes into play.
Cancer cells exploit the body’s natural angiogenic mechanisms by producing large amounts of VEGF (vascular endothelial growth factor) and other pro-angiogenic signals. These molecules stimulate surrounding blood vessels to grow new branches into the tumor mass. As a result, the tumor gains access to a dedicated blood supply, which not only fuels continued growth, but also provides a pathway for cancer cells to enter the circulation and metastasize to distant organs.
One of the hallmark features of tumor progression is the so-called “angiogenic switch”—a tipping point at which the balance of signals in the tumor microenvironment shifts decisively in favor of angiogenesis. Before this switch, the tumor remains small and dormant. But once pro-angiogenic factors like VEGF overpower anti-angiogenic controls, the tumor begins to rapidly expand and invade nearby tissues. This switch is a key step in the transition from a harmless lesion to a life-threatening cancer.
Moreover, the new blood vessels formed in tumors are often abnormal—leaky, disorganized, and poorly structured. This irregular architecture contributes to hypoxia, which further drives VEGF production and worsens tumor aggression. These abnormal vessels can also impair immune cell infiltration, helping the tumor to evade immune detection and resist therapy. Understanding tumor angiogenesis—and how cancer cells hijack this vital biological process—has been pivotal in developing new strategies to block tumor growth and spread.
Therapeutic Targeting of Angiogenesis
Anti-angiogenic therapies are a class of cancer treatments designed to starve tumors by cutting off their blood supply. Since tumors rely on angiogenesis to grow and metastasize, blocking this process has become a major strategy in oncology. The goal is to prevent the formation of new blood vessels, limit tumor oxygen and nutrient supply, and thereby inhibit further growth. One of the most well-known drugs in this category is bevacizumab (Avastin), a monoclonal antibody that targets VEGF, the key molecule that stimulates blood vessel formation. By neutralizing VEGF, bevacizumab disrupts the signal that tells blood vessels to grow into the tumor. It has been approved for several cancers, including colorectal cancer, non-small cell lung cancer, and glioblastoma.
Another group of anti-angiogenic agents includes tyrosine kinase inhibitors (TKIs) such as sunitinib and sorafenib. These drugs block the VEGF receptors on the surface of endothelial cells, preventing them from responding to VEGF signals. TKIs are commonly used in the treatment of renal cell carcinoma, hepatocellular carcinoma, and thyroid cancer. Despite their promise, anti-angiogenic therapies come with limitations. Many tumors eventually develop resistance, either by activating alternative angiogenic pathways or by adapting to low-oxygen conditions. Additionally, while these drugs can delay disease progression, they often provide only modest improvements in overall survival. Side effects such as hypertension, bleeding, poor wound healing, and proteinuria can also limit their long-term use in some patients.
Nevertheless, anti-angiogenic therapy remains a valuable option—particularly when combined with chemotherapy, immunotherapy, or radiation—and continues to be an area of active research aimed at overcoming resistance and enhancing effectiveness.
Angiogenesis in Other Diseases
Angiogenesis plays a dual role in disease—it can be either excessively activated or dangerously suppressed, depending on the condition. Understanding this balance is essential not only for treating cancer but also for managing a range of non-malignant diseases.
In several chronic disorders, excessive angiogenesis contributes to tissue damage and disease progression. For example, in diabetic retinopathy and age-related macular degeneration (AMD), abnormal new blood vessels form in the retina. These vessels are fragile and prone to leaking, leading to vision loss. Similarly, in psoriasis and rheumatoid arthritis, overactive angiogenesis supports persistent inflammation by supplying oxygen and nutrients to expanding inflammatory tissues.
On the other hand, insufficient angiogenesis can impair healing and tissue repair. In chronic wounds, such as diabetic foot ulcers, poor blood supply prevents proper regeneration. In peripheral artery disease (PAD) and ischemic heart disease, blocked arteries reduce blood flow to tissues, and a failure to stimulate adequate angiogenesis leaves these tissues starved of oxygen, contributing to pain, tissue loss, or even heart failure.
In the field of tissue engineering and regenerative medicine, angiogenesis is viewed as a key tool for success. Scientists are working to design materials and therapies that promote controlled blood vessel growth to support the survival of engineered tissues, grafts, or implants. Encouraging angiogenesis in this context is vital for delivering oxygen and nutrients to newly forming tissues, allowing them to integrate and function within the body. Thus, angiogenesis is not just a target to block in cancer—it is also a therapeutic opportunity to harness in diseases where blood supply is deficient. Balancing angiogenesis, whether through stimulation or inhibition, is increasingly recognized as a critical frontier in medicine.
You Can Watch More on OncoDaily Youtube TV
Written by Toma Oganezova, MD
FAQ
What is angiogenesis?
Angiogenesis is the process by which new blood vessels form from existing ones, crucial for tissue growth and healing.
Why is angiogenesis important in cancer?
Tumors stimulate angiogenesis to build a blood supply that supports their growth and allows metastasis.
What is VEGF and its role in angiogenesis?
VEGF (vascular endothelial growth factor) is a key molecule that promotes the formation of new blood vessels.
What are anti-angiogenic therapies?
These are treatments designed to block the formation of new blood vessels, often used to starve tumors of nutrients.
Which diseases involve abnormal angiogenesis?
Cancer, diabetic retinopathy, macular degeneration, psoriasis, and rheumatoid arthritis are linked to excessive angiogenesis.
What is the angiogenic switch in cancer?
It's a shift where tumors begin producing more pro-angiogenic factors, enabling blood vessel growth and aggressive tumor expansion.
How is angiogenesis triggered?
It is triggered by factors like hypoxia, which activates genes like VEGF through HIF-1α signaling.
What are the main types of angiogenesis regulators?
Pro-angiogenic (VEGF, FGF, PDGF) and anti-angiogenic factors (thrombospondin, angiostatin, endostatin).
What role does imaging play in angiogenesis therapy?
Imaging guides delivery of anti-angiogenic drugs and monitors treatment response in tumors.
Can angiogenesis be used for healing purposes?
Yes, stimulating angiogenesis helps treat chronic wounds and restore blood flow in ischemic conditions.
-
Challenging the Status Quo in Colorectal Cancer 2024
December 6-8, 2024
-
ESMO 2024 Congress
September 13-17, 2024
-
ASCO Annual Meeting
May 30 - June 4, 2024
-
Yvonne Award 2024
May 31, 2024
-
OncoThon 2024, Online
Feb. 15, 2024
-
Global Summit on War & Cancer 2023, Online
Dec. 14-16, 2023